Demonstration of Listeria innocua and Escherichia coli growth in ready-to-eat vegan foods
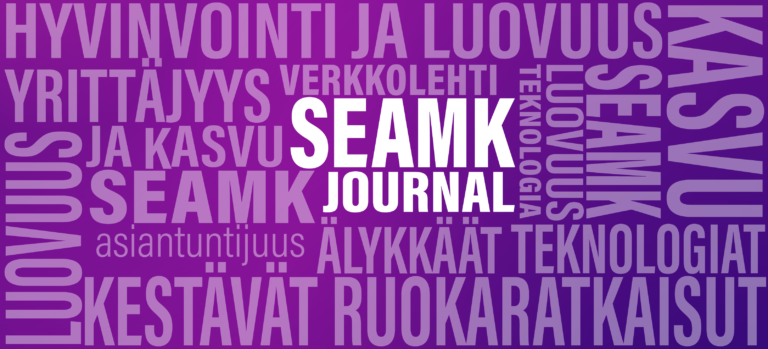
Carolin Müller, Orcid ID 0000–0003–3917–8466, Seinäjoki University of Applied Sciences, Ostwestfalen-Lippe University of Applied Sciences and Arts
Jarmo Alarinta, Orcid ID 0000-0002-0901-8472, Seinäjoki University of Applied Sciences
Björn Frahm, Orcid ID 0000–0003–4046–7804, Ostwestfalen-Lippe University of Applied Sciences and Arts
Margit Närvä, Orcid ID 0000-0002-4937-3938, Seinäjoki University of Applied Sciences
Gun Wirtanen, Orcid ID 0000-0002-5134-647X, Seinäjoki University of Applied Sciences
Suositeltu viittaus: Müller, C., Alarinta, J., Frahm, B., Närvä, M., & Wirtanen, G. (2025). Demonstration of Listeria innocua and Escherichia coli growth in ready-to-eat vegan foods. SeAMK Journal, 2, article 1. https://urn.fi/URN:NBN:fi-fe2025040223221
Abstract
Microbial food safety is the master key for reducing illness caused by the consumption of foodstuffs. This demonstration therefore aims to use non-pathogenic surrogates to draw conclusions whether vegan ready-to-eat model food serves as a base for growth of pathogenic Escherichia coli and Listeria monocytogenes in vegan ready-to-eat meat substitute in case of a potential contamination, causing a reduction of food safety.
Packages of ready-to-eat vegan meat substitutes were inoculated with certain level of non-pathogenic L. innocua and E. coli and incubated at varying environmental conditions before they were examined for changes in the physico-chemical properties.
The observed ability of the microbes to grow in the snacks may cause a reduction in food safety. This demonstration shows that these ready-to-eat-snacks may cause a reduction in food safety if the product studied is contaminated.
Keywords: Vegan food; Ready-to-eat food, RTE, Listeria innocua; Escherichia coli; food safety; microbial spoilage; shelf life study
Tiivistelmä
Mikrobiologinen elintarviketurvallisuus on tärkeää vähennettäessä elintarvikevälitteisiä sairauksia. Tämän työn tarkoituksena oli käyttää ei-patogeenisiä mikrobikorvikkeita päätelmään, toimiiko vegaaninen valmissyötäväksi tarkoitettu malliruoka, esim. lihankorvike, patogeenisten bakteerien kuten Escherichia colin ja Listeria monocytogeneksen kasvualustana ja täten mahdollisena saastumislähteenä, mikä heikentää elintarviketurvallisuutta.
Syötäväksi tarkoitettujen vegaanisten lihankorvikkeiden pakkauksiin siirrostettiin tietyt määrät ei-patogeenisia L. innocua– ja E. coli– bakteereita. Pakatut valmisruoka-annokset inkuboitiin eri ympäristöolosuhteissa ennen kuin valmisruoista tutkittiin niiden fysikaalis-kemiallisia muutoksia.
Tulosten perusteella elintarviketurvallisuus valmisruoissa heikentyi mikrobien kasvukyvyn mukaan. Tulokset osoittivat, että syötäväksi valmiit välipalat voivat heikentää elintarviketurvallisuutta, jos tuotteet ovat saastuneet säilytyksen aikana.
Avainsanat: Vegaaniset ruoat; syötäväksi valmiit elintarvikkeet, RTE-tuotteet, Listeria innocua; Escherichia coli; elintarviketurvallisuus; mikrobiologinen pilaantuminen; säilyvyystutkimus
1 Introduction
The popularity, variety and consumption of ready-to-eat foods (RTE) is increasing since they allow consumers to have a convenient and quick snack or meal, especially when under time pressure. A further increase can be expected due to growing demand for convenience food and changing lifestyles (Kotzekidou, 2016). For example, Choi (2022) investigated college students’ consumption of RTE food. He concluded that RTE food is eaten as both meals and snacks, demonstrating that the distinction between meals and snacks is no longer clear (Choi, 2022). On the other hand, a clear need for microbial food safety is required because RTE foods do not require preparation before they are consumed (Commission Regulation (EC) 2073/2005).
Moreover, the number of people adopting vegetarian or vegan diets is increasing (Agriculture and Agri-Food Canada et al., i.a.). This development explains why a wide selection of vegan food is now available, including plant-based meat and dairy substitutes (Agriculture and Agri-Food Canada et al., i.a.; Leitzmann, 2018). It also explains the increase in the consumption of vegan food (GfK & AMI, 2024).
Food safety aims to keep consumers’ health at a high level. It is based on the prevention of hazards by applying Good Manufacturing Practices (GMP), Good Hygienic Practices (GHP) and the Hazard Analysis Critical Control Points (HACCP)-system (Matissek, 2020; Lawley et al., 2012; Reij & Den Aantrekker, 2004). A hazard is defined as “a biological, chemical or physical agent in, or condition of, food or feed with the potential to cause an adverse health effect“ (Regulation (EC) 178/2002 of the European parliament and of the council 178/2002, 2002, p. 8). The occurrence of a hazard can lead to a loss of food safety, e.g. due to reduced microbial quality. But if a contamination does occur, different properties of the food may affect the microbial growth (Lawley et al., 2012; Bhat et al., 2012). These properties are either extrinsic, e.g. temperature, or intrinsic, e.g. acidity and water activity (Bhat et al., 2012). Processing e.g. thermal treatment, and implicit factors like synergism also affect the quality of the food (Bhat et al., 2012). Regarding microbial food safety, microbial cell counts can be monitored and kept at an acceptable level by taking the maximum allowed cell counts for certain microorganisms or microbes into consideration, as defined for various foodstuffs in Annex 1 of Regulation (EC) no. 2073/2005 (2005).
For many years, an increase in cases of foodborne illnesses, mainly through biological hazards, has been observable (Käferstein & Abdussalam, 1999; Yang et al., 2017). Biological hazards in food include all kinds of microbes which affect human health after the consumption of contaminated foodstuffs, e.g. the foodborne pathogens Listeria monocytogenes and enterohaemorrhagic E. coli (EHEC) (Kotzekidou, 2016; Lawley et al., 2012; Schlech & Acheson, 2000; Hunter, 2003; Orsi & Wiedmann, 2016; Bergis et al., 2021).
E. coli is a common gram-negative, non-spore-forming, aerobic bacterium with the ability to grow at low oxygen contents and which can be found in the intestinal tract of humans and other warm-blooded animals (Hunter, 2003; Odonkor & Ampofo, 2012). The occurrence of it in food-handling areas indicates faecal contamination (Odonkor & Ampofo, 2012; Torres, 2017). Foodborne, pathogenic members of this species, EHEC, are the cause of most of the infections with E. coli in Europe (Allocati et al., 2013).
Listeria is gram-positive, non-spore forming and facultatively anaerobic bacteria (Lawley et al., 2012; Orsi & Wiedmann, 2016; Bergis et al., 2021; Jemmi & Stephan, 2006; Bhunia, 2008; Mohan et al., 2019). The foodborne pathogen L. monocytogenes causes the disease listeriosis (Kotzekidou, 2016; Schlech & Acheson, 2000; Orsi & Wiedmann, 2016; Bergis et al., 2021) and can also be found in RTE food (Kotzekidou, 2016; Schlech & Acheson, 2000). L. monocytogenes infections can lead to symptoms of different severities, e.g. diarrhoea or, in the worst case, death (Matissek, 2020; Borchers et al., 2009; Schlech & Acheson, 2000).
Several studies are available, investigating the occurrence of microbial contamination in vegan meat alternatives (Barmettler et al., 2025; Magnussen, 2024; Roch et al., 2024), e.g. Magnussen (2024) studied the growth of L. monocytogenes in vegan salami whereas Barmettler et al. (2025) had a focus on highly processed alternatives like vegan nuggets, burger and schnitzel alternatives and others. All studies came to the same conclusion, that vegan meat substitutes can support microbial growth (Barmettler et al., 2025; Magnussen, 2024; Roch et al., 2024). Nevertheless, further studies are necessary to understand the source of contamination and the microbial growth in vegan products better (Roch et al., 2024).
Consequently, the aim of this investigation was to use the non-pathogenic bacteria surrogates to mimic the development of microbial growth of L. monocytogenes and pathogenic E. coli in a vegan RTE meat substitute. This is intended to demonstrate the microbial growth behaviour and the extent to which growth is progressing and impact on foodstuffs’ properties if contamination occurs to draw conclusions on the impact on food safety. It will thus be clarified whether food safety could be an issue for vegan RTE foods, depending on the storage conditions and whether measures must be taken to increase food safety.
2 Materials and methods
2.1 Vegan ready-to eat model food
The model food in focus of this demonstration is a RTE vegan meat substitute produced in Finland and available in Finnish supermarkets. The ingredients are: carrot, water, paprika, rapeseed oil, wheat flour, jalapeno, red onion, coconut oil, pea protein, rice flour, pea fibre, thickeners (modified corn starch, E 461, xanthan gum), brown sugar, spices (including paprika, garlic, chili, habanero chili, cinnamon), potato starch, salt, gelling agent locust bean gum, flavourings (including paprika), corn starch, stabilizers (guar gum, E 450), iodized salt, white pepper, acidity regulator E 500, preservative potassium sorbate, colouring agent E 160b and lime powder . The product is sold in 260 g packages. Modified atmosphere packaging (MAP) is used, meaning that an artificial gas atmosphere is created in the packages with the aim of having a preservative effect. The MAP is composed approximately as follows: ≤ 1% oxygen (O2), 30% carbon dioxide (CO2) and 70% nitrogen (N2). This model food is in scope of the current investigation as the snacks can be consumed without prior heating, i.e., the consumer is directly affected by a microbial contamination. Moreover, a vegan meat substitute was in focus of this study because many vegan meat substitutes are available on the market which are highly consumed, not just only by people following a vegetarian and vegan diet. Another reason why this product was chosen for this investigation is its composition. It is well known that meat is a proper base for microbes to growth, but it is not clear what is the impact of vegan meat substitutes on microbial growth. The model food contains several, partly natural preservatives which may inhibit microbial growth (Juneja et al., 2017).
2.2 Inocula preparation
It is well known, that the ubiquitous occurrence of microbes leads to certain possibilities of contamination of food products (Reij & Den Aantrekker, 2004; Fritsche, 2016), e.g., through ingredients (Reij & Den Aantrekker, 2004), contaminated food contact area (Käferstein & Abdussalam, 1999) and improper handling methods also affect the product quality (Reij & Den Aantrekker, 2004). The food safety of RTE food can also be endangered if the consumers do not follow good hygiene practices in e.g., storages.
As an example, the occurrence of E. coli in food-handling areas indicates faecal contamination (Odonkor & Ampofo, 2012; Torres, 2017). Foodborne, pathogenic members of this species, EHEC, are the reason for most of the infections with E. coli in whole Europe (Allocati et al., 2013). But also L. monocytogenes is known as a foodborne pathogen causing the disease listeriosis (Kotzekidou, 2016; Schlech & Acheson, 2000; Orsi & Wiedmann, 2016; Bergis et al., 2021) and can also be found in RTE food (Kotzekidou, 2016; Schlech & Acheson, 2000).
This is why in the current demonstration, packages containing the vegan snacks were artificially contaminated with either E. coli ATCC® 25922™ (Microbiologics, Inc., St. Cloud, USA) or L. innocua ATCC® 33090™ (Microbiologics) or a combination of both microbes, to mimic natural contamination by microbes (Bergis et al., 2021). Both microbes are non-pathogenic strains and are classified in risk group one (Microbiologics, 2021). The non-pathogenic strains were used for safety reasons. This demonstration chose L. innocua and non-pathogenic E. coli as surrogates for L. monocytogenes and pathogenic E. coli to mimic the pathogen’s growth because the latter ones can endanger food safety of vegan RTE snacks (Kotzekidou, 2016; Schlech & Acheson, 2000). As L. innocua has similar properties to L. monocytogenes (Orsi & Wiedmann, 2016; Bergis et al., 2021; ISO 11290-2:2017, 2017, pp. 27–28) the use of L. innocua as surrogate for L. monocytogenes is recommended in the instructions for shelf-life studies testing for L. monocytogenes in RTE food by Bergis et al. (2021).
The methods used were adapted from, the instructions for shelf-life studies testing for L. monocytogenes in RTE food drawn up by ANSES, the French agency for food, environmental and occupational health safety, and the European Union Reference Laboratory for L. monocytogenes (Bergis et al., 2021) and from several regulations by the International Standards Organization (ISO).
Each step for investigating microbial growth was performed under sterile conditions (EN ISO 7218:2007/Amd 1:2013, 2013, pp. 2–3). For artificial contamination, inocula were prepared as described in Figure 1. For preparation the inocula, one for each strain, KWIKSTIKs™ were applied to Tryptone Soy Agar (TSA) in accordance with the supplier’s instructions using the streak plate method (Microbiologics, 2021). After incubation for 48 h at 35°C, a single colony was transferred to TSA, again applying the streak plate method, followed by incubation at 37°C for 24 h (Beaufort et al., 2019; Odonkor & Ampofo, 2012; Microbiologics, 2021).To obtain liquid inocula, a single colony was then transferred to Tryptone Soy Broth (TSB), followed by incubation at the temperature targeted for the incubation of inoculated foodstuffs, which was 9°C (Beaufort et al., 2019). L. innocua was incubated for 5 days. Unlike L. innocua, E. coli did not grow at 9°C, and thus it was incubated at 37°C for 24 h. After the incubation time had elapsed, a counting chamber (Bürker-Türk, depth 0.1 mm, 0.0025 mm2) was used to determine the cell count of the inoculated TSB (Beaufort et al., 2019; Madigan et al., 2020). The cell count was then adjusted to the desired count for inoculating the model food by diluting the inoculated TSB using Maximum Recovery Diluent (MRD) as diluent (Beaufort et al., 2019; Madigan et al., 2020). TSA, TSB and MRD were purchased from (NEOGEN Corporation, Lansing, MI, USA). The obtained final inocula were used for the injection into the food packages (Bergis et al., 2021).
Figure 1. Methods for the preparation of the two inocula, one for each microorganism, and the following inoculation of the vegan snacks. The methods are based on Bergis et al., 2021, Madigan et al., 2020, Microbiologics, 2021, European Committee for Standardization, 2013 and Odonkor & Ampofo, 2012.
2.3 Sample inoculation and incubation
The microbes were applied solely or in combination using one of the following inoculation levels: 0 (reference samples), 102, 103, 104 or 105 colony forming units (cfu) per package, i.e. 0, 0.385, 3.85, 38.46, 384.62 cfu/g (Beaufort et al., 2019; Bergis et al., 2021). These inoculation levels were chosen for several reasons. As defined in regulation EC no. 2073/2005 (2005), 100 cfu/g is the max. limit for L. monocytogenes in foodstuffs available on the market during shelf life. To demonstrate the microbial growth behaviour in case of a possibly occurring contamination with < 100 cfu/g, the inoculation level 102, 103 and 104 cfu per package, i.e., 0.385, 3.85 and 38.46 cfu/g were applied. The inoculation level of 105 cfu per package, i.e. 384.62 cfu/g was applied to demonstrate a worst-case scenario.
Since samples properties are not to be affected, the volume of the added inoculum should be < 1% of the mass of the vegan snack (Beaufort et al., 2019) i.e. not more than 2.6 ml per package (Beaufort et al., 2019;). Therefore, 1 ml of an inoculum was used to inoculate the foodstuffs when the microbes alone were to be added (Beaufort et al., 2019; cf. part 3.2.1). In the case of combined application, samples were inoculated with 2 ml inoculum, 1 ml of E. coli inoculum and 1 ml L. innocua inoculum (Beaufort et al., 2019). Sterile syringes were filled with inoculum before being connected to a sterile needle which was inserted through a septum into the vegan RTE food packages (Beaufort et al., 2019).
Subsequently, the inoculum was distributed over the surface of the product by moving the needle (Beaufort et al., 2019). Afterwards, the needle was removed, the insertion hole was covered with a second septum and the snack packages were shaken carefully (Beaufort et al., 2019).
The non-inoculated samples (0 cfu/package) acted as reference samples (Beaufort et al., 2019). Non-inoculated samples were incubated unaffected. For the investigation of growth when E. coli is applied to the snacks solely, three packages per inoculation level were inoculated and tested for microbial growth whereas only two packages per inoculation level were applied for the L. innocua and combined applications. Thereafter, the packages were incubated. Storage was either refrigerated at 9°C (± 2°C) or at ambient temperature of 20°C (± 2°C) with incubation for one, three, six and nine days in closed packages. For sampling purposes, the vegan snacks were prepared in duplicate.
2.4 Investigation of gas atmosphere
When the incubation time had elapsed, the gas atmosphere in the packages was measured first, using a headspace gas analyser (Systech Instruments Ltd & Illinois Instruments, Inc.) (Bergis et al., 2021; Odonkor & Ampofo, 2012). Using the headspace gas analyser, the contents of O2, CO2 and N2 inside the packages were determined. The device was equipped with a needle that was disinfected with 70% ethanol before being inserted into the foodstuffs package without touching the RTE snack, whereupon measurement was started (Fritsche, 2016; Reij et al., 2003). After the measurement was complete, the hole caused by the needle was covered with a septum and preparation of the samples for determining microbial growth was started immediately (Fritsche, 2016; Reij et al., 2003; Beaufort et al., 2019).
2.5 Analysis of microbial growth
Beside the microbial growth of the added bacteria, the total cell count [cfu/g] of the model food was determined. To determine the L. innocua count, Harlequin® Listeria Chromogenic Agar (Ottaviani & Agosti) (LA) from NEOGEN was used (ISO 11290-2:2017, 2017, pp. 7, 9, 18); Harlequin® Tryptone Bile Glucuronide Agar (TBX) from NEOGEN was used for the E. coli count, and Tryptone Soy Agar (TSA) from NEOGEN for the total cell count. The LA and the TBX are selective agars used to verify the presence of Listeria spp. and especially L. monocytogenes in the case of LA and E. coli in the case of TBX. Using LA, the presence of L. monocytogenes can be shown and clearly differentiated from other members of Listeria spp. due to the colony’s morphology. To determine the microbial growth, approx. 215 g of the vegan RTE snacks was transferred from the packages to a sterile plastic bag (300×400 mm, thickness 50 µm, transparent, VWR International, LLC. / Avantor, Inc.) or a blender bag (plain, 3500 ml, sterile, VWR International, LLC. / Avantor, Inc.) and then was used to prepare an initial suspension and a dilution series (Beaufort et al., 2019). The remaining snacks were stored separately to be used for further testing. To prepare the initial suspension of 10-1, the samples were diluted with MRD from NEOGEN (Beaufort et al., 2019), the diluent and the foodstuff were mixed to a homogenous mash by hand for approx. 1 minute or by a laboratory paddle blender applied for 30 seconds (adapted from EN ISO 7218, 2013, pp. 4–5). When the initial suspensions were obtained, a decimal dilution series was prepared, again using MRD as diluent (Bergis et al., 2021; EN ISO 6887-1:2017, 2017, pp. 8–10, 15–17; ISO 11290-2:2017, 2017, pp. 7, 9). In consequence of the above described procedure, the detection level for microbial growth was 100 cfu/g.
Next, agar plates were inoculated with 0.1 ml of a dilution using the spread plate technique (ISO 11290-2:2017, 2017, pp. 9; EN ISO 7218:2007/Amd 1:2013, 2013, pp. 27). Except of dilution where growth as a bacterial lawn is to be expected, prepared dilutions were cultivated on agar plates in duplicate respectively. Two agar plates were inoculated with a dilution respectively. Incubation conditions were 48 h at 37°C for the Listeria count and the total cell count (ISO 11290-2:2017, 2017, pp. 9; Microbiologics, 2021). The incubation conditions for the E. coli count were 24 h at 37°C (Odonkor & Ampofo, 2012).
After the incubation, the colonies on the agar plates were counted, the average values were determined for the dilutions prepared in duplicate and microbial counts were calculated (ISO 11290-2:2017, 2017, pp.9–10; EN ISO 7218:2007/Amd 1:2013, 2013, pp. 30–31).
2.6 Investigation of physico-chemical properties
In addition to microbial growth, the snack’s physico-chemical properties – more precisely the aw value, the pH and the snack’s colour – were measured to determine if they were affected by bacterial growth. The snacks used for these determinations were mashed before the measurements were conducted. The aw value was measured using an aw measuring device (LabMaster-aw, Novasina AG, Lachen, Switzerland). The pH was measured in the mashed snack diluted 1:1 with tap water (EN ISO 7218:2007/Amd 1:2013, 2013, pp. 5–6) using a calibrated benchtop pH Meter (Orion Star™ A211 + Eutech™ ECFG7350401B pH electrode, Thermo Fisher Scientific Inc., Waltham, MA, USA). The colour evaluation was performed by image acquisition in a black box (copy stand RSX, camera arm RTX, light protector, lighting unit, Kaiser Fototechnik GmbH & Co. KG, Buchen, Germany) with a digital camera (Canon EOS 400D Digital + Canon EF-S 18-55mm, Canon INC., Tokyo, Japan). The same settings were applied for each image. The settings for the 20 g sample/image were a height of 34.2 cm, a zoom factor of 24, and two out of four light sources with a monochrome white card as the background. The subsequent colour evaluation was based on a colour histogram (Goñi & Salvadori, 2016) using ImageJ (National Institutes of Health, Bethesda, MD, USA) software developed by Wayne Rasband and colleagues (Schneider et al., 2012).
3 Results
3.1 Microbial growth
Within this investigation, it was observed that the vegan RTE snacks enable microbes to grow as can be seen in Figures 2–6. Neither Listeria spp. nor E. coli, including the pathogens L. monocytogenes and EHEC, were detected in the non-contaminated reference samples. However, cultivation on TSA revealed a naturally occurring microbial flora. With a cell count of 1.65×103 cfu/g on average one day after starting the observation, the total cell count increased to 3.28×104 cfu/g after 9 days of storage at 9°C (Figure 2). Higher total cell counts as found in the artificially contaminated vegan snacks result from the bacterial growth (Figure 2–6). The correlation coefficient describing the correlation between microbial growth and the snacks’ properties is given in Table 1.
Table 1: Correlation coefficient [-] between the mean microbial cell count determined in the snacks one, three, six and nine days after artificial contamination and mean values for pH, aw, gas atmosphere in the snacks‘ packages (O2, CO2, N2) and the snacks colour.
Taking a closer look at the artificially contaminated samples, it was observed that L. innocua can grow in the vegan snacks. However, the ability to grow in the model food is connected to the contamination level. Thus, growth was not detected within 9 days when 102 cfu/package had been added to the snacks. On the other hand, growth was observed when the snacks had been contaminated with 103 or 104 cfu/package (Figure 3). An increase in the cell count was observed reliably after six days’ storage. The highest observed average Listeria count was 1.89×103 cfu/g after nine days at 9°C in snacks inoculated with 104 cfu/package (Figure 3).
Figure 2. Development of the average total cell count [log10cfu/g] in vegan snacks: non-inoculated (black), inoculated with 103 (green) or 105 (dark blue) cfu/package Listeria innocua together with 105 cfu Escherichia coli (rhombus). Non-inoculated (black), inoculated with 103 (green) or 105 (dark blue) cfu/package E. coli together with 105 cfu L. innocua (triangle). The packages were stored at 9°C. The correlation coefficient [-] describing the correlation between microbial growth and the snacks’ properties is given in Table 1.
Figure 3. Development of the average Listeria innocua count [log10cfu/g] in vegan snacks: non-inoculated (black, circle), inoculated with 102 (pink, square), 103 (green, triangle) or 104 (dark blue, rhombus) cfu/package L. innocua. The packages were stored at 9°C. The correlation coefficient [-] describing the correlation between microbial growth and the snacks’ properties is given in Table 1.
In snacks artificially contaminated with E. coli only and stored in a refrigerated atmosphere, i.e. at 9°C, the bacteria were not detected in any sample (Figure 4). This result was obtained for each inoculation level. The bacterium was not able to grow in the snacks at this temperature level or rather the cell count remained below the detection level of 100 cfu/g. Each inoculum used for the artificial contamination of the model food was applied to TSB at both 9°C and 20°C (Beaufort et al., 2019). No E. coli growth was seen in TSB stored at 9°C. In contrast, E. coli growth was seen when TSB was stored at ambient temperature. This demonstrates that E. coli is not able to grow in a refrigerated atmosphere at 9°C. Thus, the lack of E. coli growth in the vegan RTE snacks stored at 9°C can be traced back to the environmental conditions and not to the foodstuff itself. This observation is strengthened by the fact that E. coli growth was detected in snacks artificially contaminated with 103 cfu/package and stored at an ambient temperature of 20°C with 9 days of incubation (Figure 4). After this incubation time at 20°C, the average E. coli cell count detected was 7.75×102 cfu/g, demonstrating that the snacks provide a base for E. coli to grow. The highest detected average cell counts were 7.75×102 cfu/g in closed packages and 6.68×106 cfu/g in opened packages, both stored for nine days at 20°C with an inoculation level of 103 cfu/package. In opened packages, the bacteria were already detected after 6 days of incubation whereas microbial growth in closed packages was first detected after 9 days (Figure 4). These observations show that storage in opened packages has a great impact on the microbial safety of the snacks.
Figure 4. Development of the average Escherichia coli count [log10cfu/g] in vegan snacks: non-inoculated (black, filled circle), inoculated with 102 (pink, square), 103 (light green, triangle) or 105 (dark blue, rhombus) cfu/package E. coli stored at 9°C. Snacks inoculated with 103 cfu/packages stored at 20°C in closed (mid green, triangle) and opened (dark green, triangle) packages. The correlation coefficient [-] describing the correlation between microbial growth and the snacks’ properties is given in Table 1.
Where L. innocua and E. coli were combined, both microbes behaved similarly compared to the inoculations with only single microbe species (Figures 3–5). L. innocua can grow under the given conditions, whereas E. coli cannot, leading to a decrease in cell count to below the detection limit i.e., 100 cfu/g (Figure 5). The main difference to the single application is that E. coli was detected in the snacks that had been stored at 9°C, although a decrease in cell count was observed (Figure 5). E. coli was not detected when it was applied alone (Figure 4). The highest observed cell counts after nine days of storage were 6.07×106 cfu L. innocua/g snack and 2.50×100 cfu E. coli/g snack. The inoculation levels in both cases were 105 cfu/package (Figure 5).
Figure 5. Development of Listeria innocua on LA agar (circle) and Escherichia coli on TBX agar [log10cfu/g] (square) in vegan snacks inoculated with 105 cfu/package L. innocua and various E. coli levels: non-inoculated (black), inoculated 103 (green) or 105 (dark blue) cfu/package. The correlation coefficient [-] describing the correlation between microbial growth and the snacks’ properties is given in Table 1.
When snacks were inoculated with varying levels of L. innocua in combination with fixed levels of E. coli, the E. coli was detectable on each sampling day (Figure 6). The highest E. coli count, detected after nine days of storage, was 3.41×102 cfu/g in snacks inoculated with 103 cfu L. innocua and 105 cfu E. coli. Furthermore, an increasing average L. innocua count was observed in artificially contaminated snacks during the whole storage period (Figure 6). The highest observed cell count after nine days’ storage was 4.66×105 cfu/g, which was observed in snacks inoculated with 105 cfu/package L. innocua and 105 cfu/package E. coli (Figure 6).
Figure 6. Development of Listeria innocua (LA) agar (circle) and Escherichia coli on TBX agar [log10cfu/g] (square) in vegan snacks inoculated with 105 cfu/package E. coli and various L. innocua levels: non-inoculated (black), inoculated with 103 (green) or 105 (dark blue) cfu/package. The correlation coefficient [-] describing the correlation between microbial growth and the snacks’ properties is given in Table 1.
3.2 Physico-chemical properties
The results indicated that the aw and pH were not affected by the microbes tested, regardless of the level of contamination. Environmental conditions also did not affect the foodstuffs’ properties tested (Figure 7). Both indicators remained stable over the whole storage of snacks artificially contaminated with L. innocua, with the largest changes noted being -0.4% for pH and -0.5% for aw. For non-inoculated snacks, the maximum change in average aw was a reduction by 1.1% during the nine days of storage at 9°C, while the average pH differs by max. 1.5% in the same time frame and atmosphere (Figure 7 a). Contamination with E. coli causes the average pH of the model food to increase by 2.6% at a storage temperature of 20°C, while at 9°C it decreased by 0.5% (Figure 7 b). Comparable variations were found for the average aw, more precisely by max. 0.7% when the snacks contaminated with E. coli were stored at 9°C and by 1.1% when they were stored at ambient temperature, i.e., at 20°C. Where both bacteria were present in the snacks, the average pH varied by max. -1.0%, the average aw by max. -0.3%, independent of the level of contamination (Figure 7).
The image-based determination of colour changes shows that the snack’s colour was affected neither by the growth of the single microbes nor by combinations of them. When L. innocua was present in the snacks, only slight changes in the mean colour values were observed, with reductions by 1.9% in the reference snacks and by 5.9%–6.9% in the artificially contaminated samples. The average colour value of the snacks inoculated with 102 cfu/package E. coli varied by 12.6%, whereas colour changes of 5.8%–7.0% on average were detected for snacks contaminated with other E. coli levels. Table 1 shows that the maximum variation when applying L. innocua and E. coli together was an increase by 3.8%.
Figure 7. a) Water activity (aw) and b) acidity (pH) measured in vegan snacks: non-inoculated (black), inoculated with 102 (pink), 103 (green), 104 (light green) or 105 (red) cfu/package Escherichia coli (square) or Listeria innocua (circle). E. coli 103 (light blue) or 105 (dark blue) cfu/package together with 105 cfu L. innocua (triangle). L. innocua 103 (light blue) or 105 (dark blue) cfu/package together with 105 cfu E. coli (rhombus). The correlation coefficient [-] describing the correlation between microbial growth and the snacks’ properties is given in Table 1.
4 Discussion
Controlling microbial spoilage is important to avoid reduced food safety, i.e. causing consumer illness. This is further reinforced by the observation that the vegan ready-to-eat model food allows microbes to grow. The product available on the market do not normally pose reduced food safety. However, the investigation shows that RTE snacks promote bacterial growth in the event they are contaminated.
One of many factors strengthening the observation that L. innocua, E. coli and the natural occurring flora can proliferate in the vegan snacks is the aw-value in the snacks, which was at least 0.97. This value is optimal for microbial growth in food (Bhat et al., 2012; Bergis et al., 2021; Matthews et al., 2017). Moreover, spoilage microbes can grow at aw-values as low as 0.90 (Bhat et al., 2012). The average pH of non-inoculated snacks is 5.76, which is in the range 4.1–9.4 supporting growth (Lawley et al., 2012; Bhat et al., 2012).
E. coli and L. innocua are both aerobic bacteria which also have the ability to grow at low oxygen levels (Odonkor & Ampofo, 2012; Jemmi & Stephan, 2006; Madigan et al., 2020); thus they should grow in the oxygen content of 0.3%–1.5% in the packages. It appears that microbial growth is supported by storage of the snacks in opened packages (Bhat et al., 2012; Hunter, 2003; Odonkor & Ampofo, 2012; Jemmi & Stephan, 2006).
E. coli was detectable in the vegan snack product when applied together with L. innocua. This contrasts with snacks to which only E. coli was added. Thus, a synergistic interaction possibly exists in that L. innocua supported the growth of E. coli (Bhat et al., 2012).
Observations were made which lead to the conclusion that the microbial growth is partly inhibited by the snacks (Figures 3–6). There are several possible explanations for this inhibition, including that the modified atmosphere in the snack packages, or more precisely the carbon dioxide content, as well as the average pH of 5.76 of the vegan snacks reduced microbial growth (Bhat et al., 2012; Orsi & Wiedmann, 2016). Furthermore, ingredients in the vegan snack product such as potassium sorbate and garlic impair bacterial growth due to their preservative effect (Juneja et al., 2017).
Although the snacks contain ingredients with a preservative effect, microbes can growth in the snack in case that a contamination occurs. Taking into the wide variety of vegan foodstuffs into consideration, other vegan products are likely to support the microbial growth even better due to less ingredients with a preservative effect but due to the same or better nutritional value. Additionally, modified atmosphere in packages is not available for all vegan RTE foods which will also affect the microbial growth for those foodstuffs. It can be expected that there is a higher risk for contamination and, in case that a contamination occurred, higher cell counts of aerobic bacteria in products packed in packages without modified atmosphere.
Listeria spp. is known to tolerate temperatures as low as -1.5°C (Lawley et al., 2012; Jones & D’Orazio, 2013), and therefore it is not surprising that L. innocua grows at 9°C. The absence of E. coli in the cooled snacks shows that continuous cooling improves food safety. Neither E. coli nor Listeria spp. were detected in non-inoculated snacks, and therefore the precautions applied by the supplier can be judged successful, leading to safe snacks which fulfil the requirements given in Annex 1 of Regulation EC no. 2073/2005 (2005). Storage at inappropriate temperatures supports microbial spoilage, and thus storage conditions have a great impact on food safety. Additionally, due to the increased microbial growth at ambient temperatures described in the results (cf. Figure 4), it is important that the cooling chain is not broken at any time. By keeping the snacks at refrigerated temperature, food safety can be increased.
The determination of microbial loads in reference samples, i.e. non-inoculated samples has proven, that the product available on the market meets food safety standards and do not pose a risk for consumers. Therefore, it is most likely that a contamination occurs due to improper handling of food by the customer. This may include improper storage conditions as described above and the handling with contaminated equipment (Käferstein & Abdussalam, 1999; Reij & Den Aantrekker, 2004). A main key to reduce foodborne illnesses may therefore be to raise awareness of consumers on the risks caused by improper handling of foodstuffs.
The non-pathogenic strains L. innocua and E. coli are close relatives to the pathogenic L. monocytogenes and the pathogenic E. coli, having similar properties (Orsi & Wiedmann, 2016; Bergis et al., 2021; ISO 11290-2:2017, 2017, pp. 27–28). The results of this investigation therefore indicate the growth behaviour of the pathogens. However, due to slight differences in the strains’ properties (Orsi & Wiedmann, 2016; Bergis et al., 2021; ISO 11290-2:2017, 2017, pp. 27–28), the experiments could be repeated with the pathogens themselves to obtain further information about the pathogens growth in the model food.
The investigation shows that the growth of both strains, L. innocua and E. coli, did not affect the snacks pH, aw and colour nor the gas atmosphere in the packages. However, slight various were detected over the period of the investigation. The vegan RTE snacks in the focus of this investigation do not have a homogeneous composition. The variations observed can be traced back to this inhomogeneity, among other factors.
The investigation made shows that food safety is an essential topic to protect consumers against health impairments. Although this study focussed on one product, the results can be transferred to other vegan RTE foodstuffs due to similar manufacturing process stages, similar ingredients but also as similar handling by customer can be expected which may be the main source for contaminations.
To strengthen the statements above, another study could be performed to investigate the impact of customers food handling on the microbial load and growth in vegan RTE foodstuffs. This may prove the origin of microbial contaminations and clarify the development of pathogens growth when foodstuff is handled improperly. Next, experiments can be performed to prove if an interaction between L. innocua and E. coli exist, leading to improved growth of the latter. In case that it can be proved that L. innocua can support the growth of E. coli in the snack, another study could be the investigation of the reason for the synergistic interaction. Finally, investigations on the foodstuff’s ingredients may be reasonable to prove that certain ingredients may inhibit microbial growth by their preservative effects but also to clarify if food safety can be increased by making changes in the food composition.
5 Conclusions
The investigation proved, that L. innocua and E. coli can grow in the vegan RTE model food if contamination occurs. Whereas the bacterial growth was not noticeable through changes in the aw, pH and colour of the snacks, the determination of the cell count within the model food demonstrated it. When applied unaccompanied, E. coli was not able to grow at refrigerated temperatures, i.e. at 9°C but in contrast growth was also noticeable at 9°C when L. innocua was present. This observation leads to the assumption that synergistic interactions between the two microbes exist. L. innocua can grow in the snacks when stored under refrigeration, at 9°C. This leads to the conclusion that a reduction of food safety can occur in the event of contamination after all processing steps are completed. The observations made with the non-pathogenic strains show that the risk that the food safety is affected by snacks contaminated with EHEC is present but is lower than for L. monocytogenes when storing the snacks refrigerated.
References
Agriculture and Agri-Food Canada, Appinio, Beyond Meat, Bloomberg, Civey, FAO, GfK, Global Database, Heinrich-Böll-Stiftung, IfD Allensbach, Innova Market Insights, Kantar, Lebensmittelverband Deutschland, LZ, LZ (POS-Profi-Club), Mintel, Nielsen, NielsenIQ, Oatly, … zur Mühlen Gruppe. (i.a.). Vegetarismus und Veganismus in Deutschland. Statista.
Allocati, N., Masulli, M., Alexeyev, M. F., & Di Ilio, C. (2013). Escherichia coli in Europe: An overview. International journal of environmental research and public health, 10(12), 6235–6254. https://doi.org/10.3390/ijerph10126235
Barmettler, K., Waser, S., & Stephan, R. (2025). Microbiological quality of plant-based meat-alternative products collected at retail level in Switzerland. Journal of food protection, 88(1), 100402. https://doi.org/10.1016/j.jfp.2024.100402
Beaufort, A., Bergis, H., Lardeux, A.-L., & Lombard, B. (2019). EURL Lm technical guidance document for conducting shelf-life studies on Listeria monocytogenes in ready-to-eat foods: Version 3 of 6 June 2014 – Amendment 1 of 21 February 2019. EU Reference Laboratory for Listeria monocytogenes; Anses – Food Safety Laboratory.
Bergis, H., Bonanno, L., Asséré, A., & Lombard, B. (2021). EURL Lm technical guidance document on challenge tests and durability studies for assessing shelf-life of ready-to-eat foods related to Listeria monocytogenes: Version 4 of 1 July 2021. EU Reference Laboratory for Listeria monocytogenes; Anses – Food Safety Laboratory.
Bhat, R., Alias, A.K., & Paliyath, G. (Eds.). (2012). Progress in food preservation. John Wiley & Sons.
Bhunia, A. K. (2008). Foodborne microbial pathogens – Mechanisms and pathogenesis. Springer Science+Business Media.
Borchers, A., Teuber, S. S., Keen, C. L., & Gershwin, M. E. (2009). Food safety. Clinical reviews in allergy & immunology, 39(2), 95–141. https://doi.org/10.1007/s12016-009-8176-4
Choi, J. (2022). A pilot study on RTE food purchasing and food-related behaviors of college students in an urbanized area. International journal of environmental research and public health, 19(6), 3322. https://doi.org/10.3390/ijerph19063322
Commission Regulation (EC) 2073/2005 on microbiological criteria for foodstuffs. http://data.europa.eu/eli/reg/2005/2073/oj
Fritsche, O. (2016). Mikrobiologie (Kompaktwissen Biologie). Springer Spektrum.
GfK, & AMI. (2024). Einkaufsmengen der privaten Haushalte in Deutschland an Fleischersatzprodukten von 2012 bis 2019. Statista.
Goñi, S. M., & Salvadori, V. O. (2016). Color measurement: Comparison of colorimeter vs. computer vision system. Journal of food measurement and characterization, 11(2), 538–547. https://doi.org/10.1007/s11694-016-9421-1
Hunter, P. R. (2003). Drinking water and diarrhoeal disease due to Escherichia coli. Journal of water & health, 1(2), 65–72. https://doi.org/10.2166/wh.2003.0008
International Organization for Standardization (ISO). (2013). Microbiology of food and animal feeding stuffs –. General requirements and guidance for microbiological examinations – Amendment 1, EN ISO 7218:2007/Amd 1:2013. (ISO Standard No. EN ISO 7218:2007/Amd 1:2013). https://www.iso.org/standard/52204.html
International Organization for Standardization (ISO). (2017). Microbiology of the food chain — Preparation of test samples, initial suspension and decimal dilutions for microbiological examination. Part 1: General rules for the preparation of the initial suspension and decimal dilutions. (ISO Standard No. 6887-1:2017). https://www.iso.org/standard/63335.html
International Organization for Standardization (ISO). (2017). Microbiology of the food chain – Horizontal method for the detection and enumeration of Listeria monocytogenes and of Listeria spp. – Part 2: Enumeration method. (ISO standard 11290-2:2017). https://www.iso.org/standard/60314.html
Jemmi, T., & Stephan, R. (2006). Listeria monocytogenes: food-borne pathogen and hygiene indicator. Revue scientifique et technique – Office international des epizooties, 25(2), 571–580. http://dx.doi.org/10.20506/rst.25.2.1681
Jones, G. S., & D’Orazio, S.E.F. (2013). Listeria monocytogenes: Cultivation and laboratory maintenance. Current protocols in microbiology, 31(1):9B.2.1–7. https://doi.org/10.1002/9780471729259.mc09b02s31
Juneja, V. K., Dwivedi, H. P., & Sofos, J. N. (Eds.). (2017). Microbial control and food preservation – Theory and practice. Springer Nature.
Käferstein, F., & Abdussalam, M. (1999). Food safety in the 21st century. Bulletin of the World Health Organization, 77(4), 347–351.
Kotzekidou, P. (Ed.). (2016). Food hygiene and toxicology in ready-to-eat foods. Academic Press.
Lawley, R., Curtis, L., & Davis, J. (2012). The food safety hazard guidebook. Royal Society of Chemistry.
Leitzmann, C. (2018). Veganismus, Grundlagen, Vorteile, Risiken. C.H.Beck.
Madigan, M. T., Bender, K. S., Buckley, D. H., Sattley, W. M., & Stahl, D. A. (2020). Brock Mikrobiologie. Pearson.
Magnussen, S. (2024). Growth potential for Listeria monocytogenes in vegan salami. [Masters thesis, Swedish University of Agricultural Sciences]. (Agriculture Programme – Food Science Molecular Sciences. 2024:07). Epsilon Archive for Student Projects. https://stud.epsilon.slu.se/20095/
Matissek, R. (2020). Lebensmittelsicherheit, Kontaminanten – Rückstände – Biotoxine. Springer Spektrum.
Matthews, K. R., Kniel, K. E., & Montville, T. J. (2017). Food microbiology: An introduction. (4th ed.). ASM Press.
Microbiologics. (2021). Streak plate method for colony isolation illustrated instructions. Retrieved March 31 2025 from https://www.microbiologics.com/Streak-Plate-Method-for-Colony-Isolation-Illustrated-Instructions
Mohan, V., Wibisono, R., de Hoop, L., Summers, G., & Fletcher, G. C. (2019). Identifying suitable Listeria innocua strains as surrogates for Listeria monocytogenes for horticultural products. Frontiers in microbiology, 10, 2281. https://doi.org/10.3389/fmicb.2019.02281.
Odonkor, S. T., & Ampofo, J. K. (2012). Escherichia coli as an indicator of bacteriological quality of water: an overview. Microbiological research, 4(1), 5–11, e2. https://doi.org/10.4081/mr.2013.e2
Orsi, R. H., & Wiedmann, M. (2016). Characteristics and distribution of Listeria spp., including Listeria species newly described since 2009. Applied microbiology and biotechnology,100(12), 5273–5287. https://doi.org/10.1007/s00253-016-7552-2
Regulation (EC) 178/2002 of the European parliament and of the council. Laying down the general principles and requirements of food law, establishing the European Food Safety Authority and laying down procedures in matters of food safety. http://data.europa.eu/eli/reg/2002/178/oj
Reij, M. W., & Den Aantrekker, E. D. (2004). ILSI Europe risk analysis in microbiology task force. Recontamination as a source of pathogens in processed foods. The international journal of food microbiology, 91(1), 1–11. https://doi.org/10.1016/S0168-1605(03)00295-2
Roch, F., Dzieciol, M., Quijada, N. M., Alteio, L. V., Mester, P., & Selberherr, E. (2024). Microbial community structure of plant based meat alternatives. npj science of food, 8(27). https://doi.org/10.1038/s41538-024-00269-8
Schlech, W. F., & Acheson, D. (2000). Foodborne listeriosis. Clinical infectious diseases, 31(3):770–775. https://doi.org/10.1086/513125
Schneider, C. A., Rasband, W. S., & Eliceiri, K. W. (2012). NIH image to imageJ: 25 years of image analysis. Nature methods, 9(7), 671–675. https://doi.org/10.1038/nmeth.2089
Torres, A. G. (2017). Escherichia coli diseases in Latin America—a ‘One Health’ multidisciplinary approach. Pathogens and disease, 75(2), ftx012. https://doi.org/10.1093/femspd/ftx012
Yang, S.-C., Lin, C.-H., Aljuffali, I. A., & Fang, J.-Y. (2017). Current pathogenic Escherichia coli foodborne outbreak cases and therapy development. Archives of microbiology, 199, 811–825. https://doi.org/10.1007/s00203-017-1393-y